HEAT
Heat is the activity of life – it is the atomic exchanges that make up our physical world. To consider heat as the primary motivator behind a design requires an appreciation of the properties that explain its performance. To design for heat is to design for energy.
Heat is not a property of a system. Heat is a process. It is the transfer of energy between systems. It is a registration of activity. The focus on heat and energy in architectural design is a method of redefining the syntactical exchanges that established the modern age. Thermodynamics offers a means of recharging our aesthetic culture in an era that aims to integrate science and technology into the habitation of space.
THERMODYNAMICS
In the mid-nineteenth century, a debate brewed over the concept of caloric heat as either a substance of an entity versus a property of the entity, thus giving birth to the field of thermodynamics.
Thermodynamics is the branch of physics that relates heat to work and energy. There are three primary laws and a fourth supplementary law – the Zeroth Law – that define the behaviors of thermodynamics:
First Law of Thermodynamics: Energy can neither be created or destroyed (law of conservation) and therefore the Internal Energy [U] of a system can be converted into another form of energy but cannot be destroyed. For closed systems, uninfluenced by its environment, the internal energy is given as the amount of Heat [Q] applied to a system minus the amount of Work [W] produced by the system. In this equation, the values for heat and work are path dependent (not state functions) in that they are dependent on the means for which those values are established. 1
Second Law of Thermodynamics: As energy systems evolve, the order of those systems change. The measure of these changes, and commonly considered the measure of disorder, is called Entropy [S]. In thermodynamics, the entropy of a system can never be decreased according the second law – the concept of irreversibility – and will move towards a state of equilibrium defined as the state with the maximum entropy. 1
Third Law of Thermodynamics: In the condition that a system reaches absolute zero, the entropy of that system is at its most minimal state, or also known as the embodying the residual entropy of the system, existing in its ground state. 1
Zeroth Law of Thermodynamics: When any two or more bodies are in contact with each other and are in a state of thermal equilibrium, it can be inferred that they have equality in temperature. This law is crucial to our understanding of temperature and allows for the measurement of heat transfer between two bodies – if one body is in contact with a mercury thermometer, which is then placed in contact with another body, and no change in the mercury is exhibited, then both bodies are in thermal equilibrium. 1
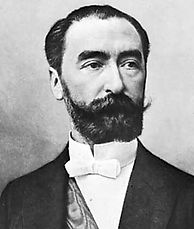
Sadi Carnot (1796-1832) - French engineer considered to be the father of thermodynamics. Through the development of his ideal heat engine, he created a new field of engineering focused on the relationship between heat and work. 2
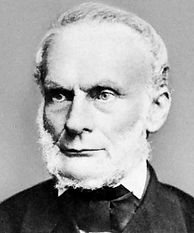
Rudolf Clausius (1822-1888) - physicist who extended work on the first law of thermodynamics and through such work defined the second law of thermodynamics in addition to the concept of entropy. 4
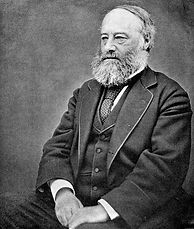
James Prescott Joule (1818-1889) - brewer and physicist who determined that the change in temperature of a medium is not contingent on the type of work done to increase the temperature, and proved that there is a direct relationship between the quantity of work and the quantity of heat produced. 3
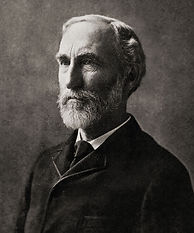
Josiah Willard Gibbs (1839-1903) - American scientist who combined the theories behind the first and second laws of thermodynamics to define the concepts of enthalpy and 'free energy' that led to the developement of "Gibbs Free Energy". 5
Energy, in its full dynamic capacity, governs the behaviors of the physical world. There are three types of energy that we experience in our daily lives – potential, gravitational, and kinetic. We are all familiar with potential and gravitational energy forms, as we have all dropped some heavy item on our foot or seen the tidal shifts of the ocean. However, it is through temperature that we become most intimate with kinetic energy.
Temperature is the average kinetic energy of an atomic system.
VIBRATIONS
Electrons of an atom are in state of constant movement around the nucleus of the atom. Energy is absorbed and emitted by the exchange between the electrons of atoms. This exchange is the kinetic energy of a system and therefore the temperature of a system.

Possible hydrogen orbitals. 6
The kinetic energy, and therefore temperature, of a system can be increased through various mechanisms. In our experience, solar energy is very effective means of warming up on a cold day. This is caused by photons bombarding an atom, exciting the movement of the electrons, escalating the interaction among the atoms, and increasing the average kinetic energy of the medium.
Radiation is one type of energy flow, in addition to conduction and convection. It is ubiquitous in our world – all objects and elements radiate into the atmosphere. As molecules are excited, their kinetic energy produces electromagnetic waves. These waves travel outwards from the source in all directions, thus the term radiation. There are two forms of radiation important to the design of our built world: shortwave radiation (visual light) is that given by the sun and contains high energy levels due to its higher frequency; and longwave radiation (infrared) which given by our surroundings due to the decomposition of atoms, and contains lower energy levels due to its lower frequency.

Diagram of electromagnetic spectrum from NASA. 7
As a material is exposed to solar radiation, the energy it receives is either transmitted, reflected, or absorbed. Transmitted energy is passed through the material with no consequence to its physical nature. The reflection and absorption of energy is dependent on the resonant frequency of the molecules that make up the material. If energy is not transmitted nor absorbed, it is reflected. Take, for example, a red apple. We see red because of all of the frequencies of energy hitting the apple, only frequency not resonating in its molecular fabric is the frequency of red, approximately 4x10^14 Hz.
When considering the material palette for thermodynamically-driven architecture, color is a simple way for isolating materials by their potential absorption and reflection of energy. Albedo is the percentage of solar energy reflected by a material. Dark materials absorb more energy, translucent materials transmit more energy, and lighter materials reflect more energy.
In architectural design, radiation is an important concept to consider in regards to the thermodynamics of the space. All objects radiate heat, especially the users who inhabit the space. Our thermal experience and thermal comfort of spaces is determined by both flows of air and by radiant heat transfer. When exposed to cooler surfaces, we feel cooler, and warmer when exposed to hot surfaces. This is caused by energy flows to sources with lower levels of energy - a balancing act to achieve thermal equilibrium. To determine the radiant heat experience by the user requires calculating the average radiant temperature exposed to the user, called the Mean Radiant Temperature (MRT) and takes into consideration the radiant temperature of a surface (Tn) and its exposure angle (Fp-n) to the inhabitant:

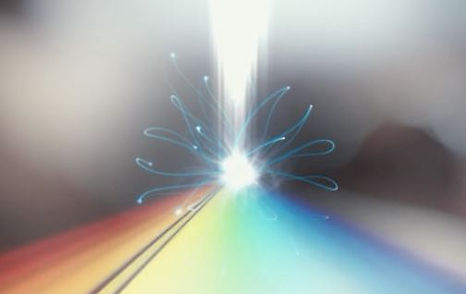
Image from Cosmos: A Spacetime Odyssey, Season 1, Ep. 5, “Hiding the Light”. Illustrating the absorption of light by a sodium atom. The black lines in the light spectrum below the atom illustrate the use of light waves by electrons to jump down into lower orbits. 8
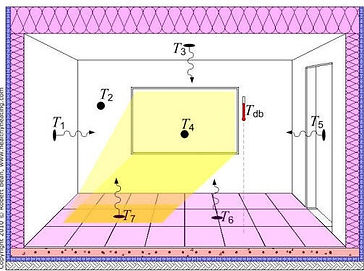
ENERGY FLOWS AND MATERIAL PROPERTIES
Once an atom absorbs solar energy and the energy is converted into kinetic energy (heat), the activity is transmitted to other molecules in proximity to that atom. The ratio of the heat energy being transferred to another molecule is called Thermal Conductivity (W/m*K) [k]. Metals are great conductors due to their molecular and atomic structures – their valence electrons can move more freely than insulators, that safeguard their electrons, and therefore conductors transfer energy through the exchange of their electrons.
Diagram showing temperature surfaces used to calculating MRT. 9
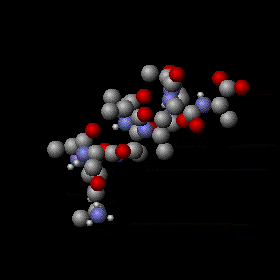
The vibrating molecule shows the motion of electrons expressing degrees of freedom in electron movement. The more movement an electron can have enables a greater amount of energy storage until the molecule breaks apart. 10
The rate at which the heat energy is transferred through a medium is called Diffusivity [α], calculated by dividing the thermal conductivity by the volumetric heat capacity of the medium. The difference between conductivity and diffusivity can be illustrated by comparing a filter to a pipe – one determines a ratio and the other the rate.
The rate of thermal diffusivity is determined not only by amount of energy flowing through a material, but also the heat capacity of the material and the density of the material. Heat Capacity (J/K) [Cp] is the amount of internal energy that a medium can absorb per increase in temperature (Kelvin, [K]) without undergoing a phase change. Some materials, such as water have high heat capacities due to their atomic structures – their electrons contain higher degrees of freedom in motion while maintaining their bonds, enabling higher internal storages of energy before increasing their temperatures or breaking apart.10
However, the physical structure of the material can also affect the heat capacity of the medium. When evaluated independent of the mass of the medium, this is called Specific Heat Capacity (J/kg*K) [cp] and when evaluated per volume of the medium – and takes into consideration the Density (kg/m2) [p] of the volume – is called Volumetric Heat Capacity (J/m3*K) [pCp] (this is not to be confused with Heat Capacity at Constant Volume [Cv] which is used mainly for comparing gases).
The distinction between heat capacity and specific heat capacity is important when considering the properties of a material. Thermodynamic properties of a system come in two forms: extensive or intensive. Extensive properties are those dependent on the amount of material of the system, and intensive properties are independent of the amount of the material of the system. For example, the mass of a cube of copper is an extensive property dependent on the size of the copper cube whereas the density of the copper cube is not dependent on the size of the cube. The heat capacity of the cube of copper is dependent on the amount of copper in the cube, whereas the specific heat capacity of the cube is only contingent on the properties of copper itself.
When selecting materials for a design the colors and textures play an important role in the haptic experience of the space. However, these experiences can be explained thermodynamically, in which the coolness of the tile floor or the warmth of cedar panels is defined by their performance at an atomic level. Thermal Effusivity is the ability of material to exchange with its thermal environment, and is conceptually the inverse to diffusivity. It is the performance of the Zeroth Law of thermodynamics in architectural design. If we place a stainless steel cube in a room with a block of oak of the same dimension in the same room, eventually the steel cube and the oak cube will reach thermal equilibrium with the air of the room. When touched, the steel cube will feel cooler than the oak cube, due to the steel's capacity to absorb the heat from the hand (high thermal conductivity and diffusivity, with high heat capacity) until a new thermal equilibrium between the hand and the cube is achieved.
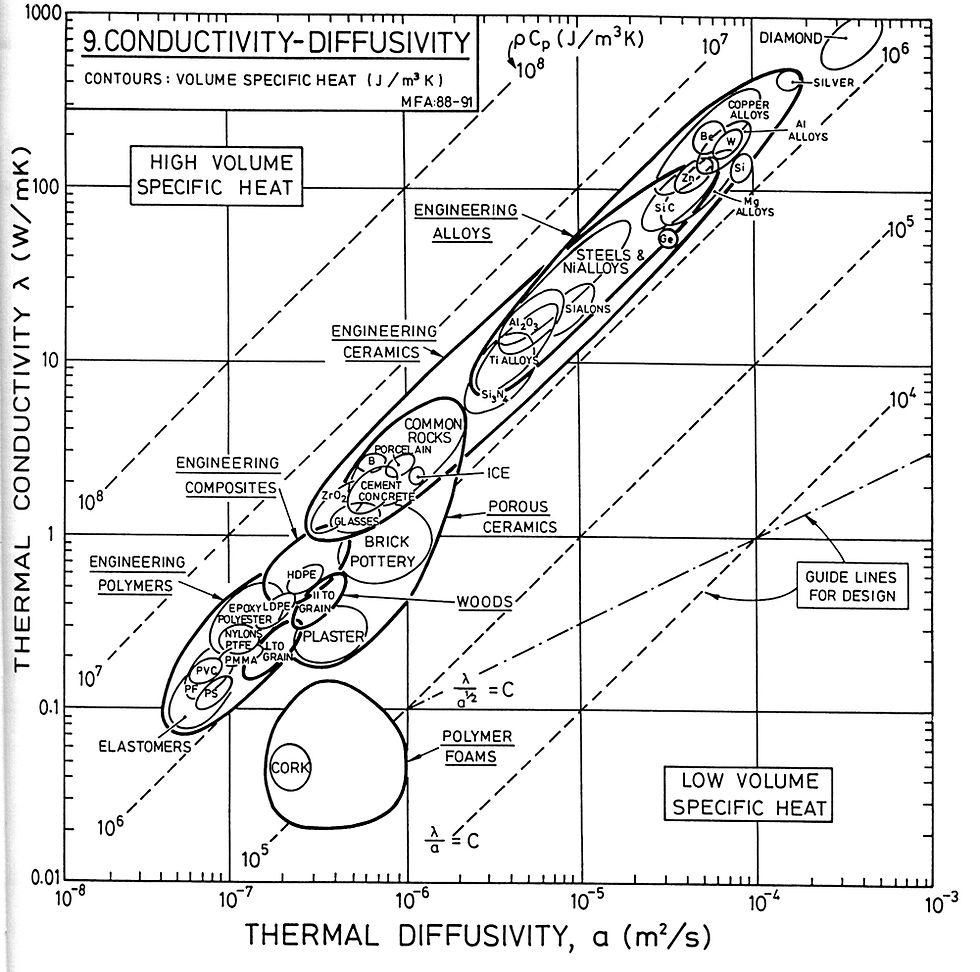
Chart showing the relationship between thermal conductivity and thermal diffusivity. 11
The physical and chemical performance of materials in design becomes salient when one considers all components of the architecture – the walls, the pipes, the inhabitants – as various media in a network of energy systems. Our world is one in constant flux, in constant movement. The design of spaces should consider this activity as a catalyst for dynamic designs, for designs that perform in conjunction with the physical phenomenon of the environment around us.
WORKS CITED
1 - “Laws of Thermodynamics.” Wikipedia, the Free Encyclopedia, January 1, 2015. http://en.wikipedia.org/w/index.php?title=Laws_of_thermodynamics&oldid=640485012.
2 - "Nicolas Léonard Sadi Carnot.”Wikipedia, the Free Encyclopedia, December 26, 2014.http://en.wikipedia.org/w/index.php?title=Nicolas_L%C3%A9onard_Sadi_Carnot&oldid=629446824.
3 - “James Prescott Joule.”Wikipedia, the Free Encyclopedia, December 26, 2014.http://en.wikipedia.org/w/index.php?title=James_Prescott_Joule&oldid=638257815.
4 - “Rudolf Clausius.” Wikipedia, the Free Encyclopedia, December 30, 2014.http://en.wikipedia.org/w/index.php?title=Rudolf_Clausius&oldid=639175995.
5 - “Josiah Willard Gibbs.”Wikipedia, the Free Encyclopedia, December 30, 2014.http://en.wikipedia.org/w/index.php?title=Josiah_Willard_Gibbs&oldid=639909955.
6 - “Hydrogen Orbitals.” Imgur. Accessed January 1, 2015.http://imgur.com/a/BG4oR.
7 - "Introduction to the Electromagnetic Spectrum." Mission Science, NASA. January 1, 2015. http://missionscience.nasa.gov/ems/01_intro.html.
8 - "Hiding the Light." Cosmos: A Spacetime Odyssey. National Geographic Channel, April 6, 2014.
9 - Robert Bean, P.L.. 'Mean Radiant Temperature | Indoor Environmental Quality With Energy Efficiency '. Healthyheating.Com. Accessed January 2 2015. http://www.healthyheating.com/Definitions/Mean%20Radiant.htm#.VKa9ICvF-So.
10 - “Heat Capacity.” Wikipedia, the Free Encyclopedia, December 26, 2014.http://en.wikipedia.org/w/index.php?title=Heat_capacity&oldid=639737190.
11 - Ashby, Michael F. Materials Selection in Mechanical Design, Fourth Edition. 4 edition. Burlington, MA: Butterworth-Heinemann, 2010.