ACTIVE BODIES
We are active beings. Whether sleeping or climbing Mt. Everest, our bodies are in constant states of flux. Through our active natures, we partake in a network of exchange with our surroundings on both a microscopic and macroscopic level. The relationship between the environment and the body is a key source in the making of place and creation of our social identities.
METABOLISM
A thermodynamic understanding of the body can begin with a simple definition of the metabolism. It is a complex process involving one’s genetics, hormones, physique, etc. Simply stated the metabolism it is the conversion of food into energy– the oxidation of calories – through a set of chemical reactions. As we age, this process ‘slows down’ in that less energy is recouped from absorbed nutrients.
A common evaluation of the metabolism is the Basal Metabolic Rate (BMR). This value is calculated under strict regulations of the environmental loads, digestion stage, activity level, etc. (This is not to be confused with the Body Mass Index (BMI) which relates one’s body weight and height. BMI remain constant whereas BMR decreases with age.)
The conversion of nutrients into energy is the essence of life. This process enables the production of not only physical work, but also the sustainability of bodily functions. All organisms are continually producing and expending energy – the human body is a thermal machine.
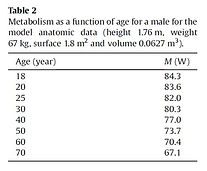
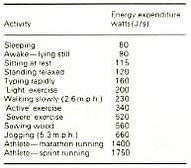
Diagram illustrating the pathways of the metabolism for living organisms. 1

Diagram illustrating the pathways of the metabolism for living organisms. 2
EXERGY
The productivity of the body as an organism is contingent on the efficient conversion of calories into energy (Metabolic Efficiency Rate), and the productive use of that energy. The energy produced by the metabolic process is used in two key thermodynamic mechanisms: energy for work and energy for heat. The differentiation between energy for work and energy for heat is established by an organism’s exergistic efficiency. Exergy is the capacity for energy to do work and metabolic exergy is the capacity for energy from food to produce work.
Exergistic efficiency of the body is therefore the ability to utilize energy for physical activities as compared to energy expended for the maintenance of homeostasis. Energy that is used for homeostasis cannot be recaptured and converted for additional work and is considered destroyed exergy, an increase in the entropy of the body’s energy cycle. The principle drive of the human body is the minimal production of entropy (Minimum Entropy Principle).
A determining factor in the exergistic efficiency of the body is the degree to which the body must mitigate external thermal conditions to the body. Thermal neutrality is the ambient temperature at which a body requires no additional energy to maintain core body temperatures, generally set at 37C or 98.7F. This temperature, like the metabolism rate, tends to change with age, increasing as we get older (we require warmer external conditions as we age).
However, this value is generalized in that the body’s thermoregulatory process is affected by more than simply ambient air temperature: the maintenance of sustainable internal conditions is contingent on a negotiation with external conditions given the physical state of the body and the production of internal heat.
THERMOREGULATION
Thermogenesis is the generation of heat of the body. Key to note is the difference between core body temperature and skin temperatures, which vary and produce the colorful thermal readings from infrared cameras (radiant heat readings of the body). At rest, the skin temperatures of an average adult male are generally around 32C-33C as compared to core temperatures at 37C, and thus evidence of the body’s constant production of heat energy. Deviations of skin temperatures from thermal neutrality temperatures help determine the thermoregulatory response of the body.
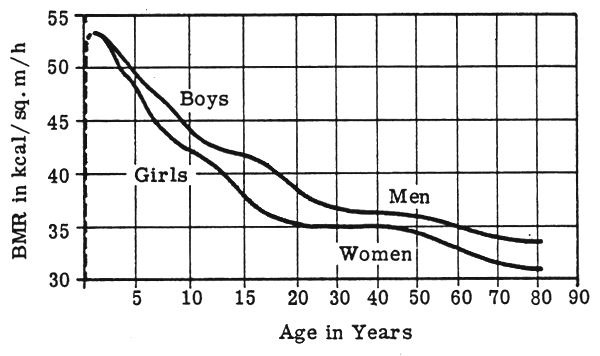

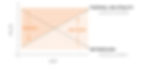
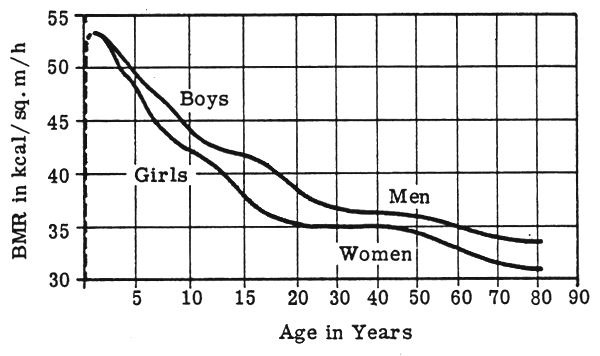
It is possible to correlate thermal neutrality and metabolic efficiency rates in regards to aging - the metabolism "slows down" and therefore less energy is availabe for the maintenance of core body temperatures and or physical work. By inhabiting spaces with warmer temperatures, the aging body is able to spend less energy. 3
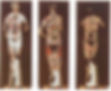
Images showing a fit male runner at 1 min, 15 mins, and 30 mins into a run. The increase in black areas in the scans indicate that the body compensates for an increase in core body temperature by sweating. The conductivity of the sweat accelerates heat loss from the body, giving the thermal reading of that area the equivalent of that of the ambient temperatures.

The thermoregulatory cycle shown here illustrates the connectivity between exterior conditions and internal notions of thermal comfort.
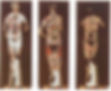
Images showing a fit male runner at 1 min, 15 mins, and 30 mins into a run. The increase in black areas in the scans indicate that the body compensates for an increase in core body temperature by sweating. The conductivity of the sweat accelerates heat loss from the body, giving the thermal reading of that area the equivalent of that of the ambient temperatures.
The maintenance of the core body temperature is managed within the brain by the hypothalamus. The skin is thermal sensor of the body, registering temperature and humidity levels of its environment. When the skin temperature deviates from the thermal neutrality temperature, the hypothalamus instructs the capillary blood vessels within the skin to compensate either through vasodilation in warm conditions (widening of the capillaries for increased blood flow, maximizing heat transfer away from the core and the activation of sweat secretion) or vasoconstriction in cold conditions (narrowing of capillaries for decreased blood flow, especially to regions of the body away from the core). Additionally, the body can engage muscle tissue to increase the temperature of the body via shivering or decrease skins temperatures through air flow around the body in motion (swinging of arms, fanning, etc).
The flow of heat from the body exists in three general forms: radiation, convection, and evaporation/respiration. The ratio of the flows is influenced by the external conditions to the body, but in general radiant heat from the body makes up 47.5% of excess heat transfer, convection at 27.5%, and evaporation/respiration at 27%.
COOLING
In addition to convective heat transfer, evaporative cooling is a primary mechanism for the removal of excess heat from the body. Our ability to sweat is essential to our endurance in hot climates. The cooling capacity of the body is the Physical Efficacy of Sweat Secretion – the ratio of heat removed from the body to the potential cooling of sweat secreted. Sweat is basically water in that its cooling capabilities derives from the molecular difference between water and air. Water is twenty-four times more conductive (at 20C) than air (at 0C). For dry skin, all excess body heat is transferred away from the body to the sweat molecules on the skin, aided by the high latent heat threshold of water and the higher conductive heat transfer between the skin and the sweat molecule than between the sweat molecule and the air (one-way flow of heat). For every gram of water that is evaporated, 153 Joules of energy are removed from the body.

4



The increase in metabolic rates is generally the same per increase in physical activity, however the differential of the metabolic rate over speed indicates that Subject1 uses more nutrients to develop the same speed as other subjects.
Internal temperatures increase at a gradual rate until stabilizing at a maximum value, only to increase again when the skin is saturated with sweat which is no longer a functional mechanism for cooling the body.
Subject1 exhibits high skin temperatures relative to internal temperatures, indicating the higher levels of the metabolism is being compensated by the increase of the convective heat transfer and the evaporation.
Due to the higher relation between the air and skin temperatures of Subject1, there is a greater exchange of energy between the body and the environment.
However, there is a limit to the evaporative cooling mechanism through sweating. The evaporative potential of sweat molecules is limited by the ability of the ambient air to absorb the added vapor from sweating. Vapor pressure, like all thermodynamic systems, moves from a high system to a low system. If the vapor pressure of the skin is higher than the ambient air, then sweat will evaporate, cooling the body. Inversely, if the vapor pressure of the skin is less than the ambient conditions, then sweat will accumulate on the skin (an issue in hot humid environments). Furthermore, when the skin is saturated with sweat (covered), the latent heat capacity of water may ‘pull heat’ into the sweat molecule, effectively increasing the thermal resistance of the skin-sweat interface.


These diagrams and graphs explain how vapor pressure is a key indicator in the sweating capacity of the body. 5
In addition to evaporative cooling, the body’s mission of heat produces a boundary layer condition that cools the surface temperatures of the skin. This is flow of air up and around the body is free confection and is determined by the difference is the skin temperature and the ambient air. But in addition to free convection, the body’s cooling process can be aided by forced convection around the body. An increase in air velocity around the skin assists in the evaporation of sweat and the conductive heat loss of the body through the movement of surrounding air molecules.
THERMAL COMFORT
The combination of air velocity, humidity levels and air temperature produce what is called the Effective Temperature. In assessing the body’s thermoregulatory system in architectural design, the building industry focuses on producing effective temperatures that minimize the influence of the environment for maximizing exergy efficiency. Practioners in the field default to the calculations that balance the many components of an inhabitant’s thermal experience of a space. The Predicted Mean Vote (PMV) was developed Povl Ole Fanger in the 70s as an evaluation of the conditions of a space. Thermal comfort surveys solicited from inhabitants evaluate spaces on a cold (-3) to hot (+3) scale. The conditions of a space – air
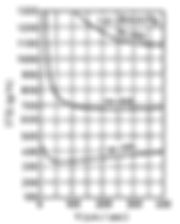
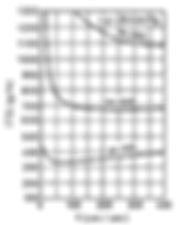
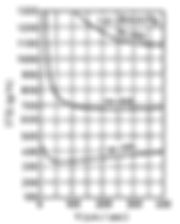
These graphs illustrate the effect of wind velocity on the heating and cooling of the body per metabolic rates. 6
temperature, mean radiant temperature, relative humidity, air speed, metabolic rate, and clothing insulation are used within a heat balance equation to determine the comfort zone within a psychometric chart. Several building scientists are advocating for a new model of comfort, one that allows for more variation and adaptability within the definition of comfort. Additionally, there is a push for the use of the Physiological Equivalent Temperature (PET) as an alternative to PMV. In PMV, skin temperatures and sweat rates are not dependent on climatic conditions. PET takes into consideration sweat rates and skin wetness, as well as other values, in the assessment of thermal conditions, however at the expense of computational time.
The use of PMV and PET is evidence of the building industry quantifying the thermal experiences of spaces that are in actuality individualistic and relative. The notion of ‘comfort’ and thermally neutral interior spaces are the result of a perspective on energy as a commodity, and legacies of climate design for the interiors of buildings. It is the onus of the designer within the architectural discipline to see the science and data behind the thermodynamics of spaces as catalysts for phenomenological and qualitative explorations of heat and the body.
WORKS CITED
1 - Clark, R. P., and Otto Gustaf Edholm. Man and His Thermal Environment. Edward Arnold, 1985.
2 - "Seed Viewer - KEGG Map". 2016.Phantome.Org. Accessed July 5 2016. http://www.phantome.org/PhageSeed/seedviewer.cgi?page=Kegg&organism=347326.2.
3 - Clark, R. P., and Otto Gustaf Edholm. Man and His Thermal Environment. Edward Arnold, 1985.
4 - Carlos Eduardo Keutenedjian Mady, Cyro Albuquerque, Tiago Lazzaretti Fernandes, Arnaldo José Hernandez, Paulo Hilário Nascimento Saldiva, Jurandir Itizo Yanagihara, Silvio de Oliveira Jr., “Exergy performance of human body under physical activities”, Energy, Volume 62, 1 December 2013, Pages 370-378.
5 - Clark, R. P., and Otto Gustaf Edholm. Man and His Thermal Environment. Edward Arnold, 1985.
6 - Clark, R. P., and Otto Gustaf Edholm. Man and His Thermal Environment. Edward Arnold, 1985.
​