FLOWS
The fundamental principle of thermodynamics is the flow of energy from higher order, like a temperature, to a lower order, having been neither created nor destroyed in the process. The design of thermodynamic architecture requires the curation of this phenomenon to manage the thermal experiences of the user.
Energy is transferred by the vibration of electrons. It flows via three mechanisms: Radiation, Conduction, and Convection. Radiation (thermal) is the emission of electromagnetic waves from a source caused by the excitement and collision of atoms within a system. Conduction is the transfer of energy through the interatomic dynamics of a body, often through the connectivity between molecules. Convection is the transfer of heat through the movement of a fluid body, such as a liquid or gas – two key agents in a building’s energy performance. Each type of energy flow is experienced differently – the sun on your back (radiation), the cool tile under your feet (conduction), or the breeze through an open window (convection) – and all three combine to produce the thermal experience that define a space.
CONVECTION CURRENTS
Of the three flow types, convection is of strong interest in the building industry. Since the early 20th century, the phenomenological ambition behind the design of our interior spaces is one of muteness. We produce hermetically sealed boxes for the maintenance of ideal thermal conditions in order to optimize human comfort.
The mechanical systems of a building, mainly the heating and cooling systems that push water and/or air through the interior spaces, occupy nearly 30% of the square footage of a building – 1/3 of architecture dedicated to the transfer of energy. Contemporary building science practices aim to develop new simulation strategies for this spatially, financially, and energy-wise taxing area of construction in order to predict pathways and values of convective heat flows through various bodies of fluids. However, these programs, computational fluid dynamics simulators (CFD analysis), are computationally expensive and tend to yield results that support expertise already gained in the field.
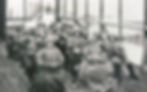
The open air movement of educational spaces in the early 20th century was an attempt to provide children with access to fresh outdoor air – concurrent with trends to open up buildings as “a prophylactic measure against tuberculosis.”
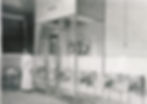
After the flu epidemic of 1918, the building became hermetically sealed and attitudes towards ventilation shifted to controlled interior climates, such as the incubators advertised by Carrier Engineering Corporation (1919). The caption to the photo reads, “Even babies can be manufactured with manufactured weather.”
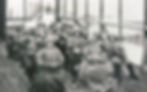
The open air movement of educational spaces in the early 20th century was an attempt to provide children with access to fresh outdoor air – concurrent with trends to open up buildings as “a prophylactic measure against tuberculosis.”
Historical approaches to climate control. 1

Example of CFD analysis of heat output from various components within a space. 2
Rather than obsess over the quantifiable dynamics of a space, which directs design towards a path of value engineering, the concept of energy flow through a building should function as a catalyst for the design of unique spaces fitted to the performance within the space – a symbiosis developed through qualitative assessment.
HEURISTIC MODELS
The three flows of energy rarely operate individually but work in conjunction to produce the effects of a space, and most importantly, the performance of a space is influence by the conditions surrounding that space. However, it is often easiest to understand the dynamics of a system by isolating a few variables and analyzing them in closed systems, unaffected by external parameters to the system. Such is the foundation for the Ideal Gas Law. Its principle equation is a way of understanding the relationships between pressure, volume, molecular concentration, and temperature within a system:

Pressure (P) is the average force of molecular enagement with a system’s boundary. In a high pressure system, the boundary walls experience a
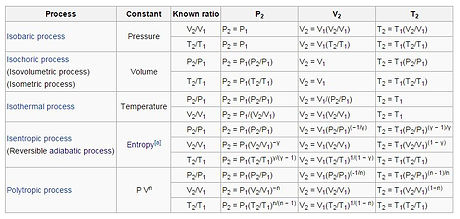
greater amount of bombardment by molecules than in a low pressure system. Therefore, a decrease in the dimensions of a system’s boundary would result in a higher pressure, assuming that no molecules were removed from the system. If the concentration of the fluid decreases, then the pressure would correspondingly decrease. Given that temperature is the average kinetic energy of a system – the average amount of vibration activity of the molecules – then an increase in temperature would produce a higher pressure or an increase in volume.
The ideal gas law is instrumental in the conceptual understanding of systems, whether they be atomic particles in a vacuum or air flows through the atrium in an office building. It allows us to compare systems or states of
Types of processes determined using the Idea Gas Law. 3
a system in establishing equilibrium to understand the dynamics of those systems. This analytic process is contingent on these systems undergoingadiabatic change – no energy is lost in the process such as conductive heat loss through the boundary of the system. Systems or states with the same volume are called isometric; the same temperature, isothermal; and the same pressure, isobaric.
HOT AIR RISING
The Convective Heat Transfer [hL] within system is a complex network of various parameters. When the temperature of a fluid increases, its kinetic energy expands the area of the molecules movement. This decreases the density of the fluid – a lighter body of gases – that rises and creates a buoyancy-driven convective heat flow. Hot air rises, not heat. The velocity of this moving fluid can provide the ventilation needed for many space types and passively cool enclosed spaces.
The generation of a convective heat flow is determined the heat flow capacities of the fluid. As a fluid encounters a heat source, such as a warm wall, the initial heat transfer to the gas is via conduction or radiation to the adjacent gas molecules. This heat is then transferred to other gas molecules via conduction and produces thermal zones, known as isotherms. The rate of heat transfer, diffusivity, determines the capacity for the conductive heat transfer of the system. Once the convective effect (decrease in density and increase in buoyancy effect) out paces the conductive heat transfer – enthalpy carried upwards is faster than enthalpy extending outwards – then the system is considered convective. The ratio between a system’s convective heat transfer to conductive heat transfer normal to the boundary is called the Nusselt number, a unitless value used to compare systems.
The flow of heat through the fluid is contingent on the heat properties of the fluid. An increase in humidity affects the conductivity (increase), diffusivity (increase), viscosity (decrease) and density (decrease) of the fluid. The Rayleigh number [Ra] is a unitless value that is the critical limit for convective versus conductive heat transfer of a fluid. It is the product of the Grashof number [Gr] (ratio of buoyancy to viscosity of a fluid) and the Prandtl number [Pr] (the ratio of momentum diffusivity and thermal diffusivity).
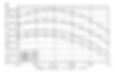


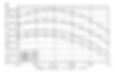
The effects of humidity on the properties of air. 4
There are two general types of convective flow: laminar and turbulent. Laminar flow (streamline) occurs when the flow of the fluid is in distinct layers without lateral mixing created by the high momentum viscosity of the fluid and tends to exist
in low velocity systems. Turbulent flow, or the production of eddies, generally occurs at higher velocities, when drag along the boundary layer is created by friction between the surface of the boundary and the fluid. The distinction between the two types can be generalized by the Reynold’s number [Re], a unitless value used to determine flow patterns in a fluid, in which high Reynold’s fluids (>5000) tend to produce turbulent flow, although not always. One of the most commonly studied types of convection currents are called Rayleigh-Benard Convection that produce the regular circulatory cells – Benard Cells – illustrated in simple convection diagrams.
CONVECTIVE ARCHITECTURE
In the performance of buildings, the convective currents can redistribute the heat within a room, therefore the location of heat sources and heat sinks is crucial to the design of thermodynamic spaces. Radiant floors are a popular contemporary technology used to add heat to a space (dry bulb temperature increase) or absorb heat from a space. However, the integration of thermally active
Animation of Rayleigh-Bernard Cells. 5
surfaces into a space can begin with windows. The Characteristic Length is the dimension that defines the scale of a physical system and in the case of windows, it is the length of the window to which the air within the space is in contact with the surface of the window. In cold climates, this value is
important when considering the convective flows of the rooms. Long tall windows provide a longer surface for cold downdrafts, as compared to strips of ribbon windows in which the laminar flow of cold air down the window is interrupted by the window sill.
The design of windows is important to the performance of spaces. But it is not only the static surfaces that affect heat flows through a room. Rarely are rooms hermetically sealed. The location of openings affects the flows of heat through the space – capturing stagnant hot or cold air, or allowing for convective circulation to distribute heat throughout spaces. This aspect is important – the location of openings has the opportunity to thermally stratify and define spaces that architecturally seem congruent – a key strategy in the design of thermodynamic architecture based on phenomenological readings of spaces.

Thermal scans of a house illustrates heat loss through windows.

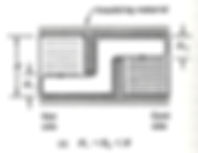


Convective flows through partitions. 6
FORCED CONVECTION
The use of buoyancy as a convective source is considered Free Convection. The circulation of air via an external source is called Forced Convection. Forced convection is crucial to the design of buildings for it is the dynamics of wind currents that push and pull the envelopes and structure of our buildings.

The main equation for determining the flow of air around buildings is the Bernoulli Equation, developed by the swiss mathematician Daniel Bernouilli in the early 18th century. The Bernoulli principle states that the pressure and velocity of a non-conductive inviscid fluid are inversely related – and
increase in one results in the decrease in the other. Like the Ideal Gas Law, the Bernoulli equation is an efficient heuristic tool for comparing systems undergoing change.
Diagram illustrating the Bernoulli Principle. 7
With regards to building design, the form of a building can directly impact the laminar flows of wind currents around a building, creating turbulent systems. These can result in inoperable windows and doors, ineffective ventilation through buildings, and structural instability.
The velocity of air through and around buildings is important to discussions of human comfort. Air movement not only redistributes heat within a space, but can actively cool the body. Additionally, the replenishment of fresh air to a space is critical to the creation of healthy spaces. However, it is important to the redefinition of thermodynamic architecture to perceive of air flow and convection as a catalyst for design.
The phenomenological reading of spaces is as important to the performance of a building as is the efficiency of an HVAC system. The flows of energy can provide a basis for a new architectural aesthetic that better integrates contemporary concerns about energy and the user.
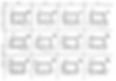
Wind diagrams illustrating the effects of opening locations. 8
- WORKS CITED
1 - Michelle Addington. “Contingent Behaviors.” Architectural Design, May–June 2009.
2 - http://www.mcc-hvac.com/home/News/Newsarchive/CFDsolvesrealworldproblems
3 - “Ideal Gas Law.” Wikipedia, the Free Encyclopedia, January 1, 2015.http://en.wikipedia.org/w/index.php?title=Ideal_gas_law&oldid=637000265.
4 - Adrian Melling, Stephan Noppenberger, Martin Still, and Holger Venzke. “Interpolation Correlations for Fluid Properties of Humid Air in the Temperature Range 100C to 200C.” Nist.gov,. 2015. Accessed January 13 2015. http://www.nist.gov/data/PDFfiles/jpcrd526.pdf.
5 - Simulation of Rayleigh-Benard-Convection, 2009.https://www.youtube.com/watch?v=nluyQThAcmM&feature=youtube_gdata_player.
6 - Bejan, Adrian author.Convection Heat Transfer. Fourth edition. Hoboken, New Jersey: Wiley, 2013, 2013.
7 - “Bernoulli’s Principle.”Wikipedia, the Free Encyclopedia, December 28, 2014.http://en.wikipedia.org/w/index.php?title=Bernoulli%27s_principle&oldid=639993799.
8 - Brown, G. Z., and Mark DeKay.Sun, Wind & Light: Architectural Design Strategies. Wiley, 2000.